Electrolocation
Electric fish can detect and localize objects in their environment using the active electric sense. This capability is called electrolocation. The mechanisms of electrolocation are similar in all electric fish, but we illustrate the principles in Apteronotus albifrons (black ghost knifefish), the fish that we study in the lab. Conceptually electrolocation involves three steps, as outlined below:
1) the fish generates an electric field around its
body
The discharge of the electric organ creates an electric field
around the fish. Since Apteronotus has a wave-type electric
organ discharge (EOD), the potential at any point in space is
constantly changing. However, we can imagine taking a "snapshot" of the
electric field at a time when the head of the fish is at its peak
positive value. If we mapped out the peak electrical potential around
the fish we would find a dipole-like field geometry, similar to the
familiar pattern of iron filings when placed near a bar magnet. The
figure below illustrates the lines of constant potential, called
isopotentials, around Apteronotus (units are in microvolts).
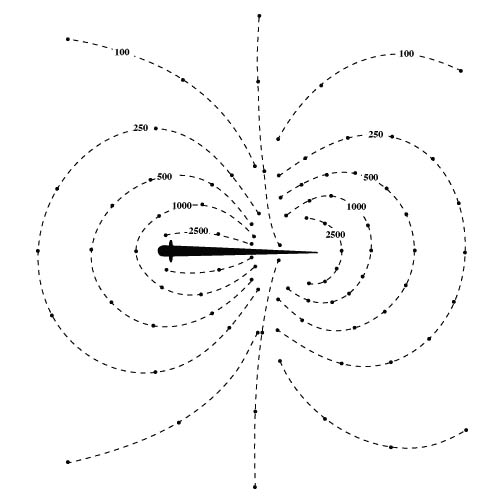
2) nearby objects in the water perturb the electric
field
Electric current flows down the voltage gradient, which means that
the lines of current flow are perpendicular to the isopotential lines
shown above. In the trunk region of the fish, the lines of electric
current flow intersect the body at approximately right angles.
When an object is brought close to the fish, it alters the pattern
of current flow. The figure below is a sketch of the lines of electric
current flow around the fish.
If the object is less conductive than the water (e.g., a rock), electric current will be shunted around the object. This will give rise to a local decrease in current density, which in turn creates an "electrical dark spot" or "electrical shadow" on the skin. If the object is more conductive than the water (e.g., a minnow), electric current will be shunted through the object because it represents a path of lower resistance. This will give rise to a local increase in current density, which in turn creates an "electrical bright spot" on the skin.
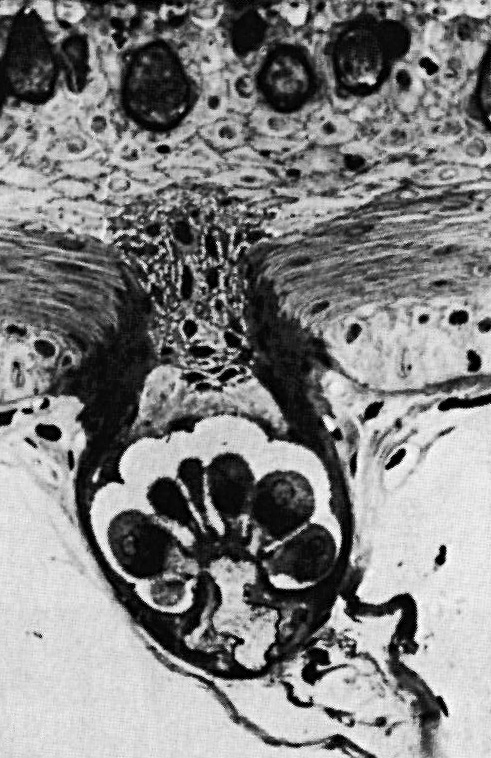
3) local perturbations are detected by electroreceptors
on the fish's body surface.
The body of Apteronotus is covered with approximately
15,000 specialized tuberous electoreceptor organs for detecting
perturbations in it's own
electric field. Each electroreceptor organ consists of a small (approx
0.1 mm diameter) pit in the skin with a cluster of sensory cells in the
bottom of the pit. The receptor cells act like miniature voltmeters and
monitor the voltage drop across the skin (the so-called transdermal
potential). The figure to the right shows a cross-sectional view of
a single tuberous electroreceptor organ. Each electroreceptor organ
gives rise to a single afferent nerve fiber which conveys sensory
information to the brain.
The electroreceptor organ density is highest in the head region of the fish (approx. 10-20 per mm2) and we sometimes think of the head as the "electrosensory fovea." The density is lower on the trunk (approx. 1-3 per mm2). On the trunk, the density is higher along the dorsal edge of the fish than on the lateral body surface. This turns out to be important because we find that prey detection typically occurs along the dorsal edge (Nelson and MacIver, 1999).
Computer reconstruction of electrosensory images
By combining the physical principles of electrosensory image formation
and our knowledge of the response properties of electrosensory afferent
nerve fibers, we can use computer models to reconstruct electrosensory
images observed by the fish during electrolocation. The figure below
shows a computer reconstructed image sequence from our prey capture
studies.